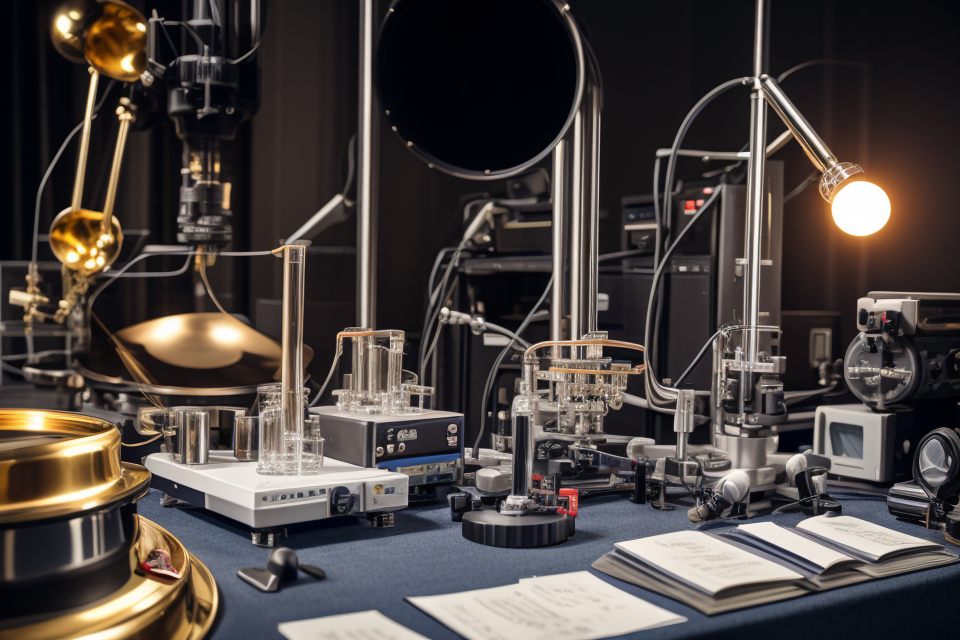
Instrumental analysis is a method of examining the physical and chemical properties of a substance to determine its composition and structure. It is an essential tool in various fields, including chemistry, biology, and pharmaceuticals. The method involves the use of advanced equipment such as spectrometers, chromatographs, and mass spectrometers to analyze samples. This article will provide an example of instrumental analysis, illustrating how it is applied in real-world scenarios. Whether you are a student or a professional, understanding the application of instrumental analysis is crucial to grasping its significance in modern science.
Instrumental analysis refers to the use of scientific instruments and techniques to analyze and measure physical and chemical properties of materials. An example of instrumental analysis is the use of a gas chromatograph to separate and analyze the components of a mixture of hydrocarbons. The gas chromatograph separates the different hydrocarbons based on their physical and chemical properties, such as boiling point and polarity, and then measures the amount of each component in the mixture. This type of analysis is commonly used in the petrochemical industry to analyze the composition of crude oil and other hydrocarbon mixtures. Other examples of instrumental analysis include titration, spectrophotometry, and high-performance liquid chromatography (HPLC).
Introduction to Instrumental Analysis
Definition of Instrumental Analysis
Instrumental analysis is a branch of analytical chemistry that deals with the quantitative and qualitative analysis of substances using specialized instruments. These instruments are designed to measure physical and chemical properties of substances, such as their density, refractive index, and optical properties. The data obtained from these measurements can be used to identify and quantify the presence of specific compounds in a sample.
Instrumental analysis involves the use of a wide range of techniques, including spectroscopy, chromatography, and mass spectrometry. These techniques are based on the interaction of electromagnetic radiation with matter, which allows the identification and quantification of different components in a sample. The choice of technique depends on the properties of the sample and the information required.
One of the key advantages of instrumental analysis is its ability to provide highly accurate and precise results. This is particularly important in fields such as pharmaceuticals, where small differences in the composition of a drug can have a significant impact on its effectiveness. In addition, instrumental analysis is often much faster and more efficient than traditional wet chemistry methods, which can be time-consuming and labor-intensive.
Overall, instrumental analysis is a powerful tool for the analysis of complex mixtures and the identification and quantification of specific compounds. Its ability to provide highly accurate and precise results, as well as its speed and efficiency, make it an essential tool in many fields of science and industry.
Importance of Instrumental Analysis
Instrumental analysis refers to the quantitative measurement of physical and chemical properties of materials. It plays a crucial role in various fields, including chemistry, biology, physics, and engineering. Here are some of the reasons why instrumental analysis is so important:
- Precision and accuracy: Instrumental analysis provides precise and accurate measurements of physical and chemical properties of materials. This helps researchers to obtain reliable and reproducible results, which are essential for scientific research.
- Time-saving: Instrumental analysis methods are often automated and can be performed quickly and efficiently. This saves time and effort compared to traditional manual methods of analysis.
- Sensitivity: Many instrumental analysis methods are highly sensitive, allowing for the detection of small changes in physical and chemical properties of materials. This is important for detecting subtle changes that may not be detectable by other methods.
- High throughput: Many instrumental analysis methods can analyze large numbers of samples simultaneously, making them ideal for high-throughput screening applications.
- Non-destructive analysis: Many instrumental analysis methods are non-destructive, meaning that they do not damage the sample being analyzed. This is important for preserving the integrity of the sample and for studying dynamic processes in real-time.
Overall, instrumental analysis is a critical tool for scientific research and has numerous applications in various fields. Its ability to provide precise, accurate, and sensitive measurements of physical and chemical properties of materials makes it an indispensable tool for scientists and researchers.
Types of Instrumental Analysis
Spectrophotometry
Spectrophotometry is a type of instrumental analysis that is used to measure the absorption or transmission of light by a substance. It is based on the principle that a substance absorbs specific wavelengths of light, and the amount of light absorbed is proportional to the concentration of the substance.
Spectrophotometry is widely used in various fields, including chemistry, biology, and physics, to determine the concentration of a substance, identify the presence of specific compounds, and study the chemical and physical properties of a substance. It is a simple, rapid, and accurate method that requires minimal sample preparation and can be performed using a variety of instruments, such as spectrophotometers, colorimeters, and spectroscopes.
In spectrophotometry, a sample is placed in a cuvette or a flask, and the light passing through the sample is measured using a light source and a detector. The absorption or transmission spectrum of the sample is then plotted against the wavelength of the light, and the peak absorption or transmission wavelength is used to determine the concentration of the substance.
There are two types of spectrophotometry: UV-Visible spectrophotometry and visible spectrophotometry. UV-Visible spectrophotometry measures the absorption of light in the ultraviolet and visible regions of the spectrum, while visible spectrophotometry measures the transmission of light in the visible region of the spectrum.
Overall, spectrophotometry is a valuable tool in instrumental analysis that provides accurate and reliable results for a wide range of applications.
Chromatography
Chromatography is a type of instrumental analysis that separates, identifies, and quantifies components in a mixture based on their interactions with a stationary phase and a mobile phase. There are several types of chromatography, including:
- Gas Chromatography (GC): This type of chromatography separates components based on their ability to vaporize and interact with a stationary phase coated with a non-volatile solid. GC is commonly used to analyze volatile and semi-volatile compounds in gases and liquids.
- Liquid Chromatography (LC): LC is a type of chromatography that separates components based on their interactions with a stationary phase and a mobile phase. There are several subtypes of LC, including:
- High-Performance Liquid Chromatography (HPLC): HPLC is a type of LC that uses a pressurized mobile phase and a narrow bore column to achieve high resolution and separation efficiency. HPLC is commonly used to analyze biological samples, pharmaceuticals, and food products.
- Ion Chromatography (IC): IC is a type of LC that separates components based on their ion exchange properties. IC is commonly used to analyze water, food, and environmental samples for anions and cations.
- Size Exclusion Chromatography (SEC): SEC is a type of LC that separates components based on their size and molecular weight. SEC is commonly used to analyze proteins, polymers, and other large molecules.
- Capillary Electrophoresis (CE): CE is a type of chromatography that separates components based on their charge and mobility in an electric field. CE is commonly used to analyze biological samples, such as DNA, RNA, and proteins.
- Supersource MAXX Accessory for Chromatography: This accessory is used in chromatography to enhance the detection and quantification of components in a mixture. The Supersource MAXX Accessory provides a stable and reproducible light source for chromatography, improving the sensitivity and accuracy of the analysis.
Overall, chromatography is a powerful tool in instrumental analysis that allows scientists to separate, identify, and quantify components in complex mixtures. Its versatility and sensitivity make it an essential tool in many fields, including pharmaceuticals, biotechnology, and environmental science.
Titration
Titration is a type of instrumental analysis that is used to determine the concentration of a known analyte in a solution by reacting it with a standardized solution of a known concentration. The process involves adding the standardized solution, called the titrant, to the analyte solution while monitoring the progress of the reaction using an indicator. The endpoint of the reaction is reached when the indicator changes color, indicating that the reaction is complete.
Titration can be used to determine the concentration of a variety of analytes, including acids, bases, and other substances. The accuracy of the results depends on the accuracy of the standardized solution and the sensitivity of the indicator used.
There are several types of titration methods, including acid-base titration, redox titration, and complexometric titration. Each method is specific to certain types of analytes and requires the use of specific indicators.
Acid-base titration is used to determine the concentration of an acid or base by reacting it with a standardized base or acid solution, respectively. The endpoint of the reaction is reached when the pH of the solution changes by a certain amount.
Redox titration is used to determine the concentration of a substance that undergoes a redox reaction, such as a metal ion. The endpoint of the reaction is reached when the current generated by the reaction reaches a maximum or minimum value.
Complexometric titration is used to determine the concentration of a complex ion by reacting it with a standardized solution of a ligand that forms a complex with the analyte. The endpoint of the reaction is reached when the absorbance of the solution changes by a certain amount.
Titration is a widely used technique in analytical chemistry and is essential for the determination of the concentration of many analytes in various fields, including pharmaceuticals, biology, and environmental science.
Applications of Instrumental Analysis
Pharmaceutical Industry
The pharmaceutical industry relies heavily on instrumental analysis for the development and production of drugs. This industry requires the precise measurement of the chemical composition and physical properties of drug compounds, as well as the detection of impurities and contaminants. Here are some examples of how instrumental analysis is used in the pharmaceutical industry:
Identification and Quantification of Drug Compounds
Instrumental analysis is used to identify and quantify drug compounds in samples. This is crucial for ensuring the quality and consistency of drug products. For example, high-performance liquid chromatography (HPLC) is used to separate and analyze the different components of a drug formulation. This allows for the accurate determination of the amount of active ingredient in a drug product.
Determination of Physical Properties
The physical properties of drug compounds, such as solubility and melting point, are important for predicting their behavior in the body and for optimizing drug formulations. Instrumental analysis can provide precise measurements of these properties. For example, differential scanning calorimetry (DSC) can be used to determine the melting point of a drug compound, which can help predict its stability and solubility in different environments.
Detection of Impurities and Contaminants
Instrumental analysis is also used to detect impurities and contaminants in drug products. This is important for ensuring the safety and efficacy of drugs. For example, mass spectrometry can be used to detect trace levels of impurities in drug samples. This allows for the identification and removal of impurities before they reach the market.
Stability Testing
Instrumental analysis is also used to monitor the stability of drug products over time. This is important for ensuring that drugs remain effective and safe throughout their shelf life. For example, HPLC can be used to monitor the degradation of drug compounds over time, allowing for the optimization of storage conditions and the development of more stable formulations.
In summary, instrumental analysis plays a critical role in the pharmaceutical industry. It allows for the precise measurement of the chemical composition and physical properties of drug compounds, as well as the detection of impurities and contaminants. This helps ensure the quality, safety, and efficacy of drugs, ultimately benefiting patient health and well-being.
Environmental Monitoring
Instrumental analysis plays a crucial role in environmental monitoring by providing rapid and accurate methods for the determination of various environmental parameters. In this context, instrumental analysis refers to the use of analytical techniques to measure the physical, chemical, and biological properties of environmental samples.
Some examples of instrumental analysis techniques used in environmental monitoring include:
- Gas Chromatography-Mass Spectrometry (GC-MS): GC-MS is a powerful analytical technique used for the detection and quantification of volatile and semi-volatile organic compounds in environmental samples. This technique is commonly used for the analysis of air, water, and soil samples.
- Inductively Coupled Plasma-Mass Spectrometry (ICP-MS): ICP-MS is a highly sensitive analytical technique used for the detection and quantification of trace metals in environmental samples. This technique is commonly used for the analysis of water, soil, and air samples.
- Liquid Chromatography-Mass Spectrometry (LC-MS): LC-MS is a versatile analytical technique used for the separation and detection of various classes of compounds in environmental samples. This technique is commonly used for the analysis of pesticides, pharmaceuticals, and other organic compounds in water and soil samples.
- Turbidity Measurement: Turbidity measurement is a simple and rapid method used for the determination of the clarity of water samples. This technique is commonly used for the monitoring of water quality in rivers, lakes, and reservoirs.
These techniques are widely used in environmental monitoring to assess the quality of air, water, and soil, and to identify potential sources of pollution. The results obtained from instrumental analysis can be used to inform policy decisions and guide the development of strategies for the protection of the environment.
Food Industry
Instrumental analysis plays a crucial role in the food industry, as it enables the evaluation of food quality and safety. The food industry uses various techniques of instrumental analysis to ensure that the food products are safe for consumption and meet the required standards.
Physical Analysis
Physical analysis techniques are used to determine the physical properties of food products, such as color, texture, and viscosity. These properties are important in determining the overall quality of the food product. Some of the physical analysis techniques used in the food industry include:
- Light Microscopy: This technique is used to examine the structure of food products at a microscopic level. It helps to identify any defects or contaminants in the food product.
- Rheology: This technique is used to measure the flow properties of food products. It helps to determine the viscosity, elasticity, and yield stress of the food product.
- Colorimetry: This technique is used to measure the color of food products. It helps to ensure that the food product has the desired color and that it is not discolored.
Chemical Analysis
Chemical analysis techniques are used to determine the chemical composition of food products, such as the presence of vitamins, minerals, and fats. These properties are important in determining the nutritional value of the food product. Some of the chemical analysis techniques used in the food industry include:
- Near-Infrared Spectroscopy: This technique is used to analyze the chemical composition of food products. It helps to determine the content of proteins, fats, and carbohydrates in the food product.
- Nuclear Magnetic Resonance Spectroscopy: This technique is used to analyze the chemical composition of food products. It helps to determine the content of water, fats, and sugars in the food product.
- Gas Chromatography: This technique is used to analyze the chemical composition of food products. It helps to determine the content of volatile compounds, such as flavor compounds, in the food product.
Microbiological Analysis
Microbiological analysis techniques are used to determine the presence of microorganisms in food products. These techniques are important in ensuring that the food product is safe for consumption. Some of the microbiological analysis techniques used in the food industry include:
- Microbial Counting: This technique is used to determine the number of microorganisms present in the food product. It helps to ensure that the food product is not contaminated with harmful microorganisms.
- Pathogen Detection: This technique is used to detect the presence of pathogens in the food product. It helps to ensure that the food product is safe for consumption.
- Spoilage Detection: This technique is used to detect the presence of spoilage organisms in the food product. It helps to ensure that the food product is of good quality and is safe for consumption.
In conclusion, instrumental analysis plays a crucial role in the food industry. It enables the evaluation of food quality and safety, ensuring that the food product is safe for consumption and meets the required standards. Physical, chemical, and microbiological analysis techniques are used to determine the physical properties, chemical composition, and microbial content of food products, respectively.
Instrumental Analysis Techniques
Spectrophotometry is a commonly used instrumental analysis technique in the field of analytical chemistry. It involves measuring the absorbance or transmittance of light by a chemical substance. The technique is based on the principle that a substance absorbs certain wavelengths of light when it is exposed to it.
There are two types of spectrophotometry: UV-Visible and IR spectrophotometry. UV-Visible spectrophotometry measures the absorbance of light in the ultraviolet and visible regions of the electromagnetic spectrum. IR spectrophotometry, on the other hand, measures the absorbance of infrared radiation.
Spectrophotometry is a widely used technique in various fields, including pharmaceuticals, biology, and environmental science. It is used to determine the concentration of a substance, identify the presence of impurities, and study chemical reactions. The technique is highly sensitive and can detect small changes in the concentration of a substance.
One of the advantages of spectrophotometry is that it is a non-destructive technique. It does not alter the chemical composition of the substance being analyzed, making it ideal for studying living cells and tissues. It is also a relatively simple and inexpensive technique to perform, making it accessible to many researchers.
However, spectrophotometry has some limitations. It is highly sensitive to water, which can interfere with the measurement of other substances. It is also limited in its ability to analyze samples that do not absorb light in the UV-Visible or IR regions of the spectrum.
Overall, spectrophotometry is a powerful tool in instrumental analysis, providing valuable information about the chemical composition and behavior of substances.
Chromatography is a technique used in instrumental analysis that separates the components of a mixture based on their interaction with a stationary phase and a mobile phase. The mobile phase is typically a liquid or gas that carries the sample through the system, while the stationary phase is a solid or liquid that remains in place. There are several types of chromatography, including:
- Gas Chromatography (GC): This type of chromatography is used to separate volatile and semi-volatile compounds from a mixture. It works by injecting the sample into a column packed with a stationary phase, such as a polydimethylsiloxane (PDMS) or a metal oxide. The mobile phase is a gas, typically helium or nitrogen, which carries the compounds through the column.
- Liquid Chromatography (LC): This type of chromatography is used to separate non-volatile compounds from a mixture. It works by injecting the sample into a column packed with a stationary phase, such as silica or polymeric beads. The mobile phase is a liquid, such as water or acetonitrile, which carries the compounds through the column.
- Ion Chromatography (IC): This type of chromatography is used to separate ionic compounds from a mixture. It works by injecting the sample into a column packed with a stationary phase, such as a polymeric resin or a silica gel. The mobile phase is a solution of a particular ion, such as hydrogen ions or potassium ions, which interacts with the ionic compounds in the sample.
- Size Exclusion Chromatography (SEC): This type of chromatography is used to separate compounds based on their size. It works by injecting the sample into a column packed with a stationary phase, such as a polymeric resin or a gel. The mobile phase is a solvent, such as water or acetonitrile, which carries the compounds through the column.
In each case, the separation of the compounds is based on their interactions with the stationary and mobile phases. The separated compounds are then detected and quantified using various techniques, such as ultraviolet (UV) spectroscopy or mass spectrometry (MS). Chromatography is a powerful tool in instrumental analysis and is widely used in many fields, including pharmaceuticals, environmental science, and food science.
Titration is a common example of instrumental analysis in chemistry. It is a quantitative analytical technique used to determine the concentration of a solution by adding a known volume of a standard solution of a titrant with a known concentration to a sample solution. The reaction between the titrant and the sample is monitored until the endpoint is reached, which is the point at which the reaction is complete.
There are several types of titrations, including acid-base titrations, redox titrations, and complexometric titrations. Each type of titration is used to analyze a specific type of compound or reaction.
Acid-base titrations are used to determine the concentration of an acid or base by adding a base or acid solution of known concentration to the sample. The endpoint is reached when the pH of the solution changes due to the neutralization of the acid or base.
Redox titrations are used to determine the concentration of a redox species, such as a metal ion or a radical, by adding a redox agent of known concentration to the sample. The endpoint is reached when the redox reaction is complete.
Complexometric titrations are used to determine the concentration of a complex ion by adding a complexing agent of known concentration to the sample. The endpoint is reached when the complex ion is completely complexed.
Titration is a useful and versatile technique in analytical chemistry and is widely used in various fields, including pharmaceuticals, biotechnology, and environmental science.
Advantages and Limitations of Instrumental Analysis Techniques
Instrumental analysis techniques are widely used in chemistry and other scientific fields for the detection and quantification of various analytes. These techniques have numerous advantages and limitations that must be considered when selecting the appropriate method for a particular analysis.
Advantages:
- High sensitivity and selectivity: Instrumental analysis techniques often have high sensitivity and selectivity, allowing for the detection of trace levels of analytes in complex matrices.
- Rapid analysis: Many instrumental analysis techniques can provide results in a matter of seconds or minutes, allowing for rapid screening and identification of analytes.
- Automation: Many instrumental analysis techniques can be automated, reducing the need for manual labor and increasing throughput.
- High precision and accuracy: Instrumental analysis techniques can provide highly precise and accurate results, reducing the potential for human error.
Limitations:
- High cost: Instrumental analysis techniques can be expensive to purchase and maintain, limiting their accessibility in some settings.
- Specialized training: Many instrumental analysis techniques require specialized training and expertise to operate, limiting their use in some settings.
- Interference from matrix effects: Instrumental analysis techniques can be susceptible to interference from matrix effects, such as the presence of interfering compounds or the presence of different phases in a sample.
- Limited range of detection: Some instrumental analysis techniques may have a limited range of detection, limiting their usefulness for certain types of analytes.
In summary, instrumental analysis techniques have numerous advantages, including high sensitivity and selectivity, rapid analysis, automation, and high precision and accuracy. However, they also have limitations, including high cost, specialized training requirements, susceptibility to matrix effects, and limited range of detection. It is important to carefully consider these factors when selecting the appropriate method for a particular analysis.
Future Advancements in Instrumental Analysis
In recent years, there have been significant advancements in instrumental analysis techniques that have greatly enhanced the accuracy and efficiency of various chemical and biological tests. These advancements have enabled researchers to analyze samples more quickly and with greater sensitivity, which has led to numerous breakthroughs in fields such as medicine, environmental science, and materials science.
One area where instrumental analysis has seen significant advancements is in the development of new spectroscopic techniques. For example, surface-enhanced Raman spectroscopy (SERS) has been developed, which allows for the detection of molecules at very low concentrations by amplifying their Raman signal using a metal surface. This technique has been used to detect biomolecules such as proteins and DNA, and has potential applications in biosensors and medical diagnostics.
Another area where instrumental analysis has advanced is in the development of new chromatographic techniques. For example, two-dimensional gas chromatography (2D-GC) has been developed, which separates mixtures based on both their chemical composition and physical properties. This technique has been used to analyze complex environmental samples such as air and water, and has potential applications in the detection of pollutants and toxic compounds.
In addition to these advancements, there has been a growing interest in the development of portable and user-friendly instrumental analysis devices. These devices are designed to be used in the field or in resource-limited settings, and can provide real-time analysis of samples without the need for complex equipment or specialized training. Examples of such devices include portable mass spectrometers and handheld Raman spectrometers, which have been used in a variety of applications such as environmental monitoring and medical diagnosis.
Overall, the future of instrumental analysis looks promising, with ongoing research and development leading to new and innovative techniques for chemical and biological analysis. These advancements have the potential to revolutionize a wide range of fields and improve our understanding of the world around us.
Recap of Instrumental Analysis
Instrumental analysis is a type of analytical chemistry that involves the use of experimental techniques to identify, quantify, and analyze the physical and chemical properties of a substance. It is an essential tool in the fields of science and engineering, as it provides a way to measure the properties of materials with high accuracy and precision.
Instrumental analysis techniques can be broadly classified into two categories:
- Spectroscopic techniques: These techniques use electromagnetic radiation to probe the properties of a substance. Examples include ultraviolet-visible spectroscopy, infrared spectroscopy, and nuclear magnetic resonance spectroscopy.
- Chromatographic techniques: These techniques separate the components of a mixture based on their physical or chemical properties. Examples include gas chromatography, liquid chromatography, and capillary electrophoresis.
Instrumental analysis techniques are widely used in various fields, including pharmaceuticals, biotechnology, environmental science, and food science. They are also used in quality control and product development in various industries.
Importance of Instrumental Analysis in Different Fields
Instrumental analysis is a vital tool in various fields such as chemistry, biology, pharmaceuticals, and food science. It involves the use of sophisticated instruments to determine the physical and chemical properties of substances.
Chemistry
In chemistry, instrumental analysis is used to identify and quantify the components of a mixture. It is also used to study the kinetics and thermodynamics of chemical reactions.
Biology
In biology, instrumental analysis is used to study the composition and structure of biological molecules such as proteins, nucleic acids, and lipids. It is also used to study the interactions between biological molecules and their environment.
Pharmaceuticals
In the pharmaceutical industry, instrumental analysis is used to develop and test new drugs. It is also used to ensure the quality and purity of drugs and other pharmaceutical products.
Food Science
In food science, instrumental analysis is used to study the composition and structure of food materials. It is also used to ensure the safety and quality of food products.
Overall, instrumental analysis is an essential tool in various fields that requires precise and accurate measurements. It enables researchers to gain a deeper understanding of the properties and behavior of substances, which is crucial for the development of new technologies and products.
Final Thoughts on Instrumental Analysis
Instrumental analysis refers to the use of instruments to analyze and measure physical, chemical, and biological properties of a sample. This technique is widely used in various fields, including chemistry, biology, physics, and engineering. Some examples of instrumental analysis techniques include spectroscopy, chromatography, and mass spectrometry.
One of the main advantages of instrumental analysis is its ability to provide accurate and precise measurements. This is especially important in fields such as medicine, where accurate measurements can mean the difference between life and death. In addition, instrumental analysis can be used to analyze complex samples, such as biological tissues, which would be difficult to analyze using traditional methods.
However, instrumental analysis can also have some limitations. For example, some techniques may require expensive equipment or specialized training to operate. In addition, some techniques may be limited in their ability to analyze certain types of samples. Therefore, it is important to carefully consider the strengths and limitations of each technique before choosing which one to use.
Despite these limitations, instrumental analysis continues to be an important tool in many fields. As technology continues to advance, it is likely that new and improved instrumental analysis techniques will be developed, making it possible to analyze samples in even greater detail.
FAQs
1. What is instrumental analysis?
Instrumental analysis refers to the use of scientific instruments and techniques to analyze the chemical, physical, and biological properties of a sample. This method is commonly used in various fields such as chemistry, biology, pharmaceuticals, and environmental science to gain insight into the composition and properties of a substance. Instrumental analysis provides precise and accurate results compared to traditional analytical methods, making it an essential tool in research and development.
2. What are some examples of instrumental analysis techniques?
There are many examples of instrumental analysis techniques, including chromatography, spectroscopy, titration, and mass spectrometry. Chromatography separates the components of a mixture based on their physical or chemical properties, while spectroscopy measures the interaction between matter and electromagnetic radiation. Titration is used to determine the concentration of a substance by reacting it with a known quantity of another substance, and mass spectrometry identifies and quantifies the components of a sample based on their mass-to-charge ratio.
3. How is instrumental analysis different from other analytical methods?
Instrumental analysis differs from other analytical methods in terms of its accuracy, precision, and speed. Traditional methods often rely on manual procedures and subjective interpretation, leading to potential errors and variability. In contrast, instrumental analysis uses precise instruments and objective measurements, resulting in more accurate and reproducible data. Additionally, instrumental analysis can be performed much faster than traditional methods, allowing for more efficient analysis of large datasets.
4. What are some applications of instrumental analysis?
Instrumental analysis has a wide range of applications in various fields. In chemistry, it is used to identify and quantify the components of a sample, characterize materials, and study chemical reactions. In biology, it is used to analyze DNA, proteins, and other biomolecules, as well as to study cellular processes. In pharmaceuticals, it is used to develop and test new drugs, while in environmental science, it is used to monitor air and water quality and study the effects of pollution. Overall, instrumental analysis plays a crucial role in advancing our understanding of the world around us.